Earlier this year, climate activists held a funeral for the Okjökull glacier. Its tombstone, the first of its kind, lies on top of a volcano in Iceland, where the ice sheet has been melting at an unprecedented rate. Indeed, if all the ice in Iceland melts, it could raise sea levels around the world about about five feet. And by the end of this century, as more glaciers recede and melt, sea levels could rise by two full metres. That’s about the height of a door.
Recently, Scott Kulp and Benjamin Strauss, two climate scientists from Climate Central, an American nonprofit organisation dedicated to climate research, identified areas that are most vulnerable to projected sea-level rise by 2050 and 2100. They released maps showing parts of Mumbai, Chennai and Kolkata, and other South Asian cities, going under water in the next three decades, suggesting around 300 million people are vulnerable if humankind continues to emit more carbon.
This is about three-times as many people as estimated to be vulnerable in an older model working with the same emission trends.
The significant mismatch arises from how scientists assess land elevation. A more precise prediction requires more precise elevation data. In the early 2000s, scientists obtained the numbers from NASA’s Shuttle Radar Topography Mission (SRTM) to approximate flood risk in coastal areas. “But SRTM data has flaws,” Kulp told The Wire. The technology can’t distinguish trees from buildings and other land features, so the reported average elevation of an area can be vastly different from reality. Errors could run as high as 2 m in low-lying coastal areas.
A more precise way to calculate land elevation is by using light detection and ranging (LIDAR). In a LIDAR-based land survey, scientists attach a laser to a plane, drone or a helicopter and beam light to the ground. The reflected signal indicates how the land rises and falls as the aerial vehicle moves over it. On the flip side, LIDAR is costly and so most Asian countries – whose economies are more vulnerable to vagaries of the climate – don’t have LIDAR data.
Also read: The Struggle to Track Global Sea Level Rise
To work around this, Kulp and Strauss used machine learning. They trained a neural network to identify flaws in SRTM data for the US coast by comparing it with more than 50 million LIDAR data points from the same area. Once the network had ‘learnt’ how SRTM and LIDAR data matched up, they converted SRTM data from around the world into more precise digital elevation data, called coastal DEM, where they estimated the errors were of the order of 10 cm or less.
And when the duo overlaid the sea-level rise model onto the coastal DEM, they found that older models had underestimated flood risk. According to the coastal DEM, they found that without coastal defences, 360 million people would be vulnerable to flooding by 2100 if sea levels continued to rise as the models predict.
Kulp and Strauss also stressed that this would be the case even if all countries stuck to the terms of the Paris Arrangement. If economies don’t cut back on carbon emissions and if the Antarctic ice sheet starts to wear off, 480 million people will become vulnerable to flooding by 2100.
The carbon dioxide that we release into the atmosphere traps heat, driving global warming heating. Historical data shows that global sea levels have been rising more quickly since humankind started burning coal and petroleum as fuels. Between 1900 and 2000, the average sea level rose by about 1.7 mm each year. After 2000, it jumped to about 3 mm/year. Scientists recorded a similar trend for the Indian Ocean – except in the Northern Bay of Bengal, where sea levels rose by 5 mm/year between 1948 and 2010 (as estimated in 2015).
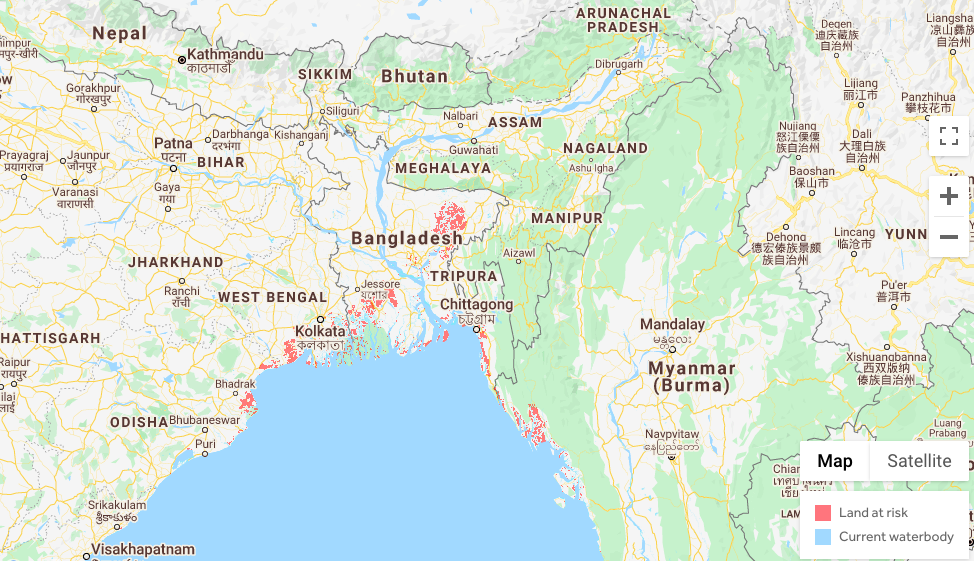
A section of the map showing parts of land at risk in a ‘bad luck’ scenario by 2050. Map: Climate Central
But the claim that certain neighbourhoods within cities could submerge doesn’t hold water.
According to the maps published along with the duo’s paper, many areas in Mumbai, Kolkata and Chennai could go under by 2100. However, many of these areas also appear to be about 8-10 m above sea level.
Roxy Mathew Koll, a climate scientist at the Indian Institute of Tropical Meteorology, Pune, agrees that the new land elevation estimates are better on a global scale and that there could be uncertainties in the data for Indian cities. “Generally, the uncertainty is above 1 meter, which is larger than the estimates of sea-level rise. But to be certain, India needs a better risk assessment analysis for its coastline,” he said.
Kulp agreed that coastal DEM is more accurate over larger areas than in smaller ones, like cities versus neighbourhoods. He said this is why scientists are talking about flood risk on global and national scales (instead of about the fates of Trombay, Besant Nagar or Siripuram).
These predictions are also limited by the fact that they don’t account for embankments and defence structures that governments have erected to protect the coast from high tide lines. According to the paper, about 110 million people in the world and about 17 million people in India are already living below these lines.
Also read: Bengal’s Diamond Harbour Records Faster Sea-Level Rise Than Other Indian Ports
But Kulp flips the threat on its head to find a silver lining: “Our results give hope that living with high-tide lines is doable” – although if the sea level rises further, more people could be exposed to extreme storms and flooding.
For example, some parts of Mumbai have always been at or below sea level, so a typical worst-case scenario when the sea level is higher would be floods due to extreme rains at high tide. “Such events, called compound events, have the potential to submerge large parts of Mumbai, at least for several days,” Koll said.
And if seawater moves further inland, it can damage soil considered useful for agricultural and even facilitate the spread of disease. In 2015, doctors in Bangladesh were able to associate the spread of cholera with sea-water intrusion. In another analysis published the same year, scientists reported that “suitable areas for Vibrio cholerae” – the bacterium that causes cholera in humans – are “predicted to increase under future climate”, including in coastal parts of eastern India and Latin America.
“Through this study, we want to put pressure on economies and countries to take note of the problem and understand the importance of coastal elevation data,” Kulp said. He, and others, argue such data will help countries assess risk more realistically and plan better for extreme events.
“Another way to look at the study is to realise that cutting back on emissions will help three-times more people,” he added. “And that investing in green technology is three-times more justifiable.”
Sarah Iqbal is a freelance science writer.