It is a truism that basic research leads to technological and economic progress. Governments and the public have come to see all of science through the lens of applications. This is short-sighted: in a democracy, science is a public good for a multitude of reasons.
The astronomer and author Carl Sagan spoke of science as a candle in the dark: a way to push back ignorance and uncertainty, a way to discover truths about our world and chart our way forward.
In the wake of the Bhopal gas leak of 1984, reacting to the horror of the most deadly industrial disaster in history, a collection of grassroots groups across India assembled to talk about the future. These groups, some of whom had existed for decades, were dedicated to spreading awareness of science and its fruits, in schools and town halls, through street theatre performances and in vernacular media. Their members, mainly non-scientists, were driven by conscience and idealism. They saw a role for science in the literacy and anti-superstition efforts of the era, but also knew the limits of a science divorced from society.
In 1988, they came together to form the All India People’s Science Network, perhaps unique in the world in its reach and depth. The network continues to be active today, teaching and popularising science, mobilising thousands of people in cities and villages, intervening in public discussions about issues ranging from genetic modification to forest loss.
This is one way in which the flame of science burns in contemporary India. Yet it’s not the aspect we usually talk about.
§
Stories about Indian science tend to focus on big-bang contributions. We’re told about the ancient invention of zero, the linguistics of Panini, the medical treatises of Charaka and the astronomical calculations of Aryabhata. A parallel technological narrative runs from ancient textiles and rustproof metalwork to modern armaments such as the Mysorean rockets used by Tipu Sultan against British East India Company forces. We celebrate the work of Srinivasa Ramanujan, J.C. Bose and C. V. Raman in British India.
Stories of science in independent India are no different. The Green Revolution of the 1960s, which increased India’s agricultural capacity manifold, made M.S. Swaminathan and Norman Borlaug household names. India’s pride at being able to loft spacecraft to Earth, Moon and Mars orbit has made heroes of the men behind the Indian Space Research Organisation, Vikram Sarabhai and Satish Dhawan. A.P.J. Abdul Kalam was India’s “Missile Man” and later the president of the country. Homi Bhabha was revered throughout India’s scientific and political establishment. It was through his efforts that the country eventually joined the club of nuclear powers in 1974.
But these singular achievements are not universally celebrated. The genesis of the All India People’s Science Network echoed the traumatic experiences of a previous generation, when the Hiroshima bomb triggered mass movements against the proliferation of nuclear weapons. The Green Revolution has all but petered out: growth in agricultural yield is slowing, India’s farmland is increasingly too saline to be usable, and the total cultivable area is dropping.
The country now faces irreversible environmental degradation and loss of wildlife, a water crisis with no solution in sight, and massive displacements of people, all as a consequence of the post-independence push towards industrialisation. This is the people’s history of Indian science, and it stands in direct contrast to the great-man narrative.
Panini is known as the “father of linguistics”; Aryabhata, the “father of astronomy”; Swaminathan, the “father of the Green Revolution”; Sarabhai, the “father of India’s space program”; and Bhabha, the “father of India’s nuclear program”. Indian science has many fathers – but no mothers to speak of and a billion neglected children.
§
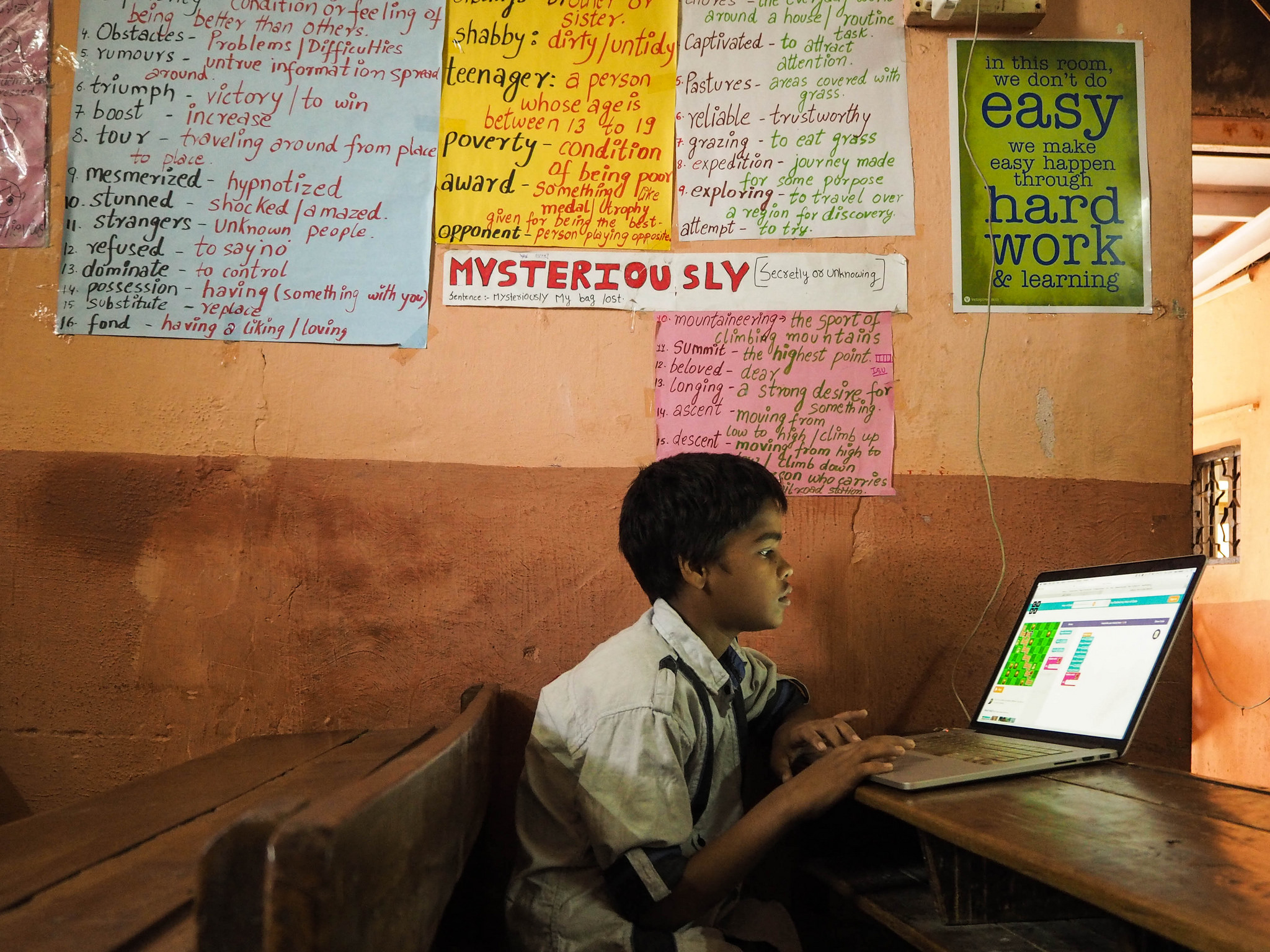
Credit: Lau Rey/Flickr, CC BY-NC 2.0
Why does India support science as a publicly-funded enterprise? The country’s total expenditure on research, including contributions from industry, has for years held steady at about 0.7% of GDP according to the Indian government’s Economic Survey of 2018. This is much lower than the 2-3% of GDP that China, the US or Germany spend. However, India’s rate of public investment in research is 0.5% of GDP, comparable to that of more wealthy countries.
Investments on this scale can only be politically justified if they are targeted toward areas of national importance, such as defence, agriculture and health. What about the argument for public investment in more basic research? This is often based on Vannevar Bush’s 1945 report to the US government, ‘Science, the Endless Frontier’. Bush knew that the Manhattan Project and other major scientific achievements of the US war effort relied on apparently useless discoveries of earlier decades. He argued that basic research would yield sustained technological and economic dividends, and therefore should be supported by public funds.
Enlightenment science in the West, with its curiosity-driven ideal, was conducted by a small set of men who enjoyed the patronage of the wealthy or the monarchy. It later borrowed the trappings of academic rigour from philosophers and historians: practices such as the sharing of work in learned societies, peer review of research findings, and formal apprenticeship of students in universities. During the Industrial Revolution, science continued within the walls of academia while technology progressed through the labours of practical men in the outside world.
However, as scientific predictions became more reliable and therefore useful to the process of invention, science and technology started to intertwine. This process culminated in the massive projects of World War II, giving us radar, transistors, computers, atomic energy and Bush’s fateful social contract. The hyphenation of science and technology as S&T has never been reversed since. In the post-war era, governments have become the single largest funders of science across the world.
In newly-independent India, science was practiced within universities, in the new engineering-centric teaching institutions such as the Indian Institutes of Technology (IITs), in the application-oriented laboratories of the Council of Scientific and Industrial Research (CSIR), and in basic research institutes such as the Indian Institute of Science (IISc) and the Tata Institute of Fundamental Research (TIFR). Some of these, including most of the universities, had existed prior to Indian independence. Each had developed within its own unique circumstances and context.
But these separate histories soon began to be erased as the administration of science and education in India moved inexorably towards uniformity. Since academic positions enjoyed relatively stable funding, science grew professionalised. It became a viable and sought-after career path for increasing numbers of people. In exchange for this stability, scientists ceded control of the research agenda. National missions such as weather forecasting, agriculture, the atomic program, myriad massive engineering projects and the expansion of India’s human resources, set the direction for India’s growing scientific cadre.
Now, after seven decades of public investment, the government is asking what has been achieved.
§
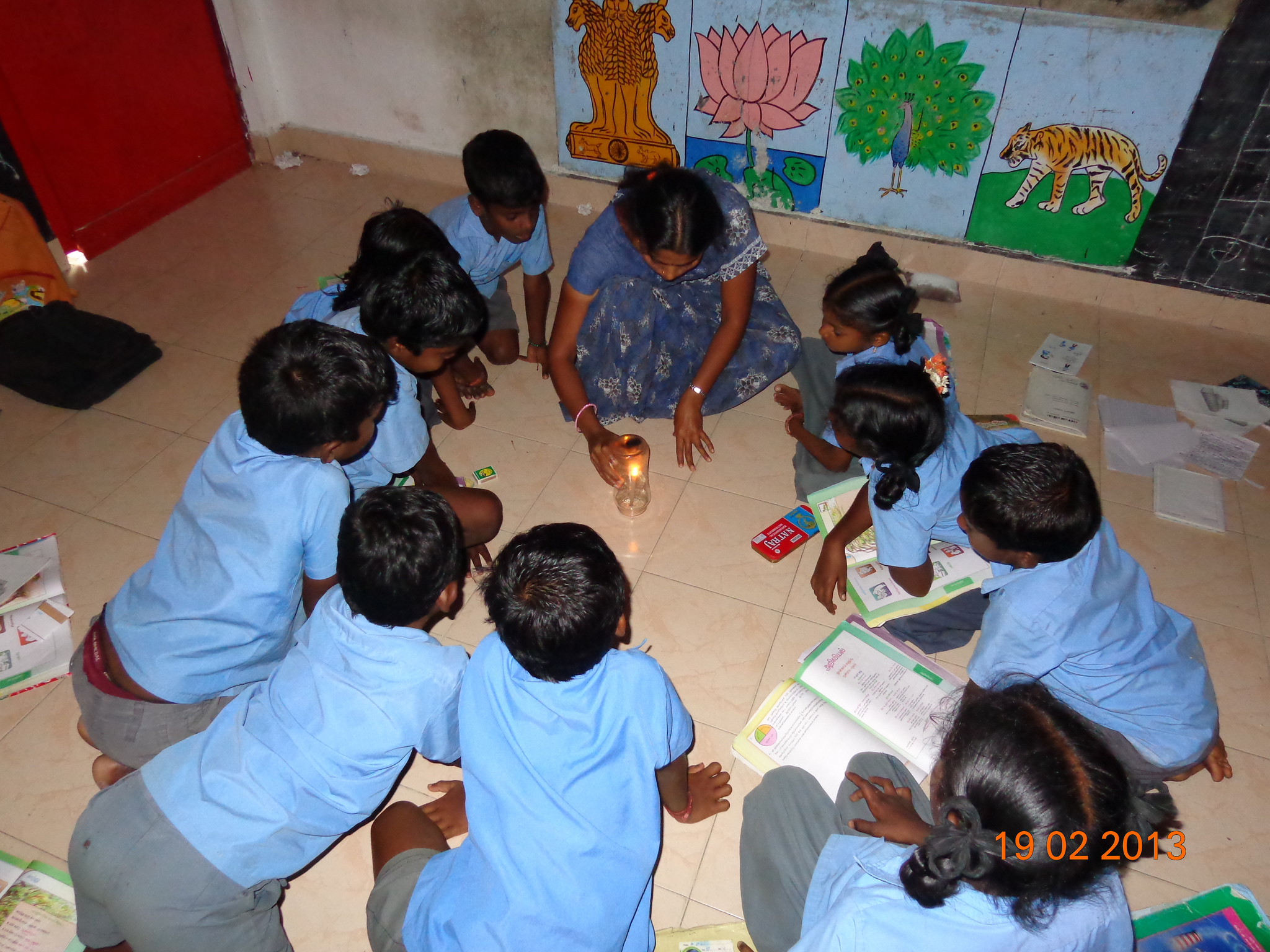
Credit: Global Partnership for Education/Flickr, CC BY-NC-ND 2.0
February 28, the anniversary of the day C.V. Raman discovered the effect for which he was awarded the Nobel Prize, is celebrated as India’s National Science Day. Writing in the Hindustan Times on the occasion this year, K. VijayRaghavan, now India’s principal scientific advisor, made the case for public investment in “blue skies” research. VijayRaghavan, an accomplished basic scientist himself and the former director of the National Centre for Biological Sciences, Bengaluru, echoed Vannevar Bush as he wrote about the benefits accrued from curiosity-driven research in India: Shambhu Nath De’s work on cholera toxin and G.N. Ramachandran’s seminal contributions to structural biology. He argued that much more could be expected if the right investments, incentives and institutional environments were put in place.
Unfortunately, this narrative starts from the premise that the only justification for public funding of science is the promise of eventual applications. This gives a flawed impression of the way science works, creates unrealistic expectations and setting funders at odds with researchers. Major Indian science funding agencies, including the CSIR, the Department of Science and Technology, the Department of Biotechnology and the Department of Atomic Energy, are under pressure to deliver on applications. Basic-research scientists are forced to shelter behind Bush’s fragile syllogism: “Our collective work may not be useful now, but history tells us it will be someday; my own work is not useful now, so there is a chance it might be someday.”
Eventually that ‘someday’ becomes today. Judged by the very yardsticks scientists themselves have put forward, Indian science has done little for the Indian people.
The Indian scientific establishment can no longer take unquestioning public support for granted. The case of the India-based Neutrino Observatory (INO) is revealing. In development for nearly two decades by a consortium of institutions including TIFR, the INO is a proposed detector shielded deep within a mountain to study the properties of fundamental particles called neutrinos. The project has a strong scientific justification, raises no safety concerns and has recently been granted an environmental clearance. Yet the effort has been dogged by claims that it will affect human health and harm forest and farm lands. Though the INO team has worked closely with the people who live around the mountain and nearby forested areas, they are accused of ignoring the sentiments of the local community.
False rumours spread faster than attempts by scientists to address them. Why do these stories have so much traction? Why is it so easy to paint scientists in a bad light? Sadly, the INO is a victim of previous failures in which precisely these kinds of lapses did occur: in which scientists ignored environmental issues or local sentiments. Such concerns are not restricted to India. The Thirty-Meter Telescope proposed to be built on Mauna Kea in Hawaii has met with strong protests from native Hawaiians who feel it would violate one of their most sacred spaces.
Across the world, public spending on esoteric scientific projects has always faced resistance, not just from the people but also from politicians. In 1969, Robert Wilson, the first director of Fermilab, was asked by a US Congressional Committee whether his expensive particle accelerator had any security applications. He replied: “It has nothing to do with defending our country, except to make it worth defending”. Wilson was arguing that there are deep and important reasons to fund science, beyond its much-touted capacity to generate technological progress. The science-for-applications framework was articulated through negotiations between the scientific community and the government, each side driven by its own narrow and self-serving logic. It’s time to ask people – not scientists, not the government but the people – why, if at all, science makes a positive contribution to their lives.
Contrast the slow but steady growth of the grassroots All India People’s Science Network with the precipitous decline of India’s government-supported universities and the under-performance of its research establishment. The longevity of the network is the result of many factors: the drive and dedication of its members, who see science as an instrument of broader change; the diversity of its activities, bubbling up from the preoccupations and motivations of its various constituencies; the diffuseness of its structure, each of its sub-groups having grown organically within a local context. I believe there are valuable lessons here on how to reimagine science in India, a deeply-rooted science worthy of public support.
What kind of science would this be?
§
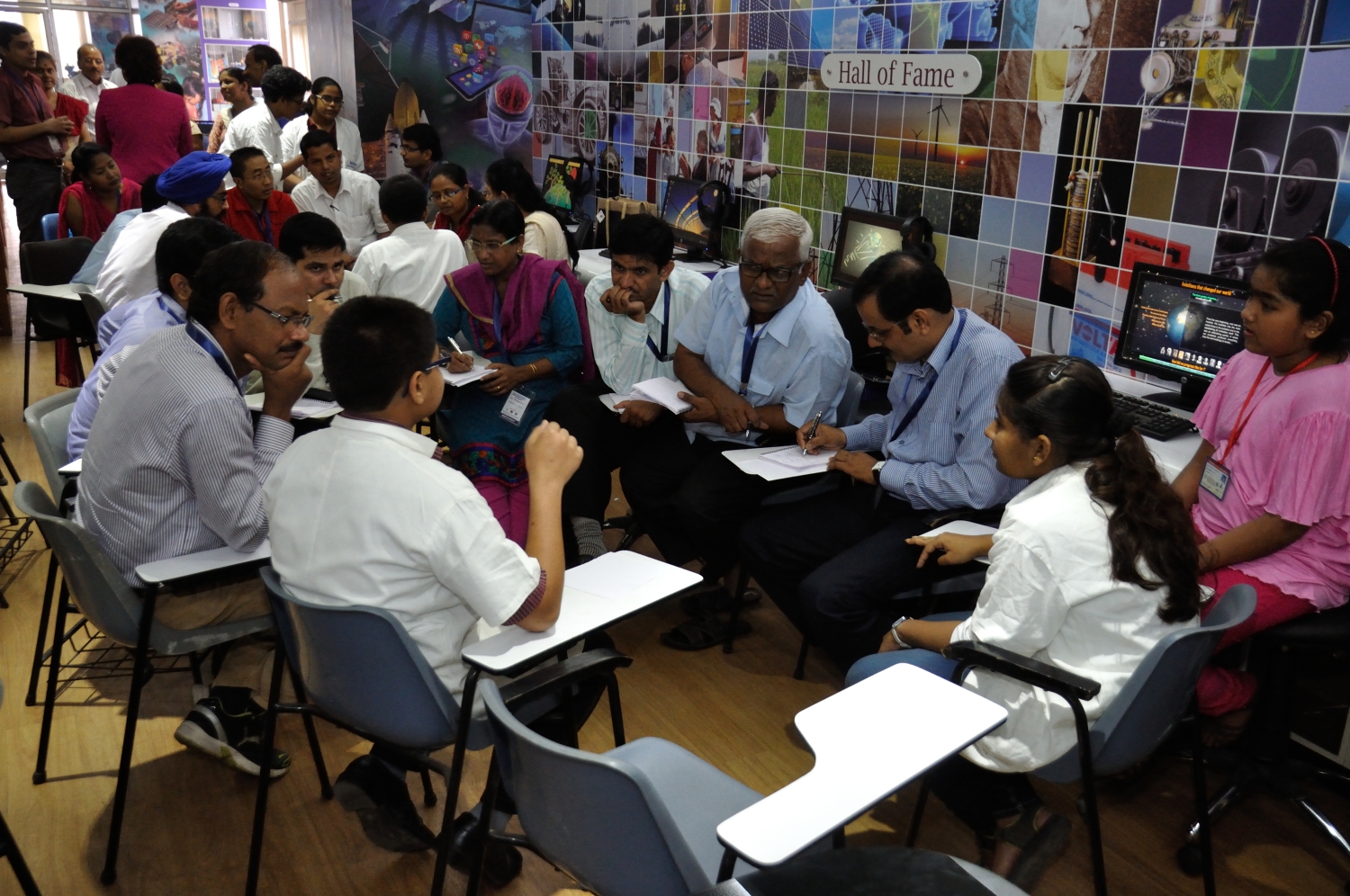
Credit: Biswarup Ganguly/Wikimedia Commons
A science that inspires. There is a strong case to fund science for the same reason we fund the arts or sport. Science is a cultural activity: it reveals unexpected beauty in the everyday; it captures the imagination of children; it attempts to answer some of humanity’s biggest questions about where we came from. Moreover, scientific ideas can be a potent component of the process by which society arrives at collective decisions about the future. Among the strongest reasons a resource-limited country such as India should fund curiosity-driven science is that the nature of future crises cannot be predicted.
It is impossible to micromanage the long-term research agenda, so the only hope is to cast a wide net. A broad and deep scientific community is a valuable resource that can be called upon to give its inputs on a variety of issues. They cannot be expected to always deliver a solution but can be expected to provide the best possible information available at any time. In this consultative process, it is crucially important to not privilege scientific experts over other participants in the discussion.
A diverse and democratic science. Science thrives within a diversity of questions and methods, a diversity of institutional environments, and a diversity of personal experiences of individual scientists. In the modern era, the practice of science has moved to a more democratic mode, away from the idea of lone geniuses and towards a collective effort of creating hypotheses and sharing results. Any tendency toward uniformity and career professionalisation dilutes and ultimately destroys this diversity. As historian of science Dhruv Raina describes it, a science that is vulnerable to the “pressures of government” is “no longer an open frontier of critical activity”. Instead, science must become “social and reflexive”.
Ideas and themes must bubble up from the broadest possible community. In India, access to such a process is limited by the accident of one’s mother tongue and social class, and this must change. Anyone who wants to should have the opportunity to understand what scientists are doing. Ultimately, this must involve not only scientists but also social scientists, historians, philosophers, artists and communicators – and the public at large.
A science that is locally rooted. Is there such a thing as an “Indian way” of doing science? Science in the abstract is said to transcend national boundaries. In practice it is strongly influenced by local experiences and local history. Unfortunately, even as national missions have faded to the background, they have been replaced by an imitation of Western fashions. It has become common to look to high-profile journals and conferences as arbiters of questions worth asking. This must stop. The key to revitalising Indian science is the careful choice of rich questions. These questions could be driven by new national missions that bring the excitement of a collective effort. Or they could be inspired by observing the complex interactions of the world immediately around us.
There is a great deal of scholarship and scientific inquiry that can arise from the study of India’s traditional knowledge systems. The country’s enormous biodiversity and human genetic diversity are an exciting and bottomless source of scientific puzzles and important secrets. Such questions would allow for a deeper two-way engagement with India’s people. This is not to say Indian scientists cannot work on internationally important problems – quite the opposite. The scientific community in India, working within their own unique contexts, could become the source of important problems that anyone in the world would be excited to work on.
A science that builds global connections. The internationalisation of science is an important goal in and of itself. While it stimulates cross-fertilisation of ideas and pushes up standards within science, it also creates opportunities for broader global discussions and engagements. The unfortunate hurdles which curtail the ability of Indian academics and students to travel abroad, and the enormous difficulty foreign academics face in obtaining necessary permissions to visit their colleagues in India, serve no purpose. In spite of all this, there is a healthy trend towards stronger international links.
Major global science funding agencies such as the Wellcome Trust and European Molecular Biology Organisation directly fund research within India. And while India’s current capacity to train its young scientists is slowly improving, Indian students are exposed to excellent opportunities abroad. The US National Science Foundation estimates there are nearly 9,000 Indian students enrolled in science and engineering PhD programs in the US alone, with thousands more spread across the world. This is a substantial fraction of the 76,000 students presently enrolled in such programs in India, according to the Ministry of Human Resource Development’s 2017 Survey.
Young Indian scholars abroad represent India to the world. They build links to productive academic and research networks, are trained in cutting edge disciplines and generate new scientific output – all while maintaining close ties to home.
A science that renews itself and passes on its values. Academic scientists have long played dual roles as teachers and researchers. Within India, science has a remarkably broad appeal. Public science talks are standing-room-only affairs, and famous scientists receive the kind of adulation typically reserved for movie stars. Students across the country are excited about science. Many aspire to become scientists themselves.
Historically, engineering and medical colleges have attracted scientifically-minded students, but this is changing. The Indian Institutes of Science Education and Research have now been running undergraduate programs for over a decade in cities across India. These institutions are to science what the IITs are to engineering, attracting some of the brightest students each year. Science programs within public universities have not fared as well, and must seize every opportunity to reinvent themselves. A science curriculum based not on dry facts but on the history and process of discovery can form the base of a broad education, in conjunction with the humanities and the arts.
§
These are just some of the reasons I believe science in India deserves public support. Every so often, the work of basic scientists has led to useful applications. But there are enough instances in which actual harm has been done in the name of science. We cannot be so naïve as to claim innocence; we must take some responsibility for this and participate fully in correcting it. This does not mean overturning our lives and institutional structures.
For a start, it means we must be open to ideas and criticism, sensitive to the consequences of our work, more integrally connected to the complex society around us. Words from the 1983 essay ‘Toward a People’s Science Movement’ by historian Mahesh Rangarajan and other remain relevant today: “Science and technology has been getting alienated from the people, their understanding and knowledge, life experiences and problems.”
It is time that every Indian, and people everywhere, are able to carry the candle of science in a way that brings meaning to each of their lives.
This essay was originally written for a forthcoming publication of the Lakshmi Mittal South Asia Institute at Harvard University, focusing on science and South Asia.
Mukund Thattai is a member of the faculty of the National Centre for Biological Sciences, a part of the Tata Institute of Fundamental Research. His research focuses on the evolution of complex cells. The views expressed here are his own. He can be reached by email and on Twitter.